Battery manufacturers are seeking chemistries that balance performance, cost, and sustainability. Enter Lithium Iron Phosphate (LFP) batteries.
Welcome to round two of my Watt Happens Next series, this time, we’re diving into how LFP is making waves in the battery world with its mix of lower costs, sustainability benefits, and shifting supply chain dynamics.
For years, high-nickel cathodes like Nickel Manganese Cobalt (NMC) and Nickel Cobalt Aluminium (NCA) reigned supreme, valued for their superior energy density. But the tide has turned. The rising costs of nickel and cobalt, combined with supply chain vulnerabilities, have prompted a shift toward LFP.
By eliminating cobalt and leveraging more abundant raw materials, LFP presents a more sustainable and socially responsible option crucial for manufacturers navigating tightening regulations.
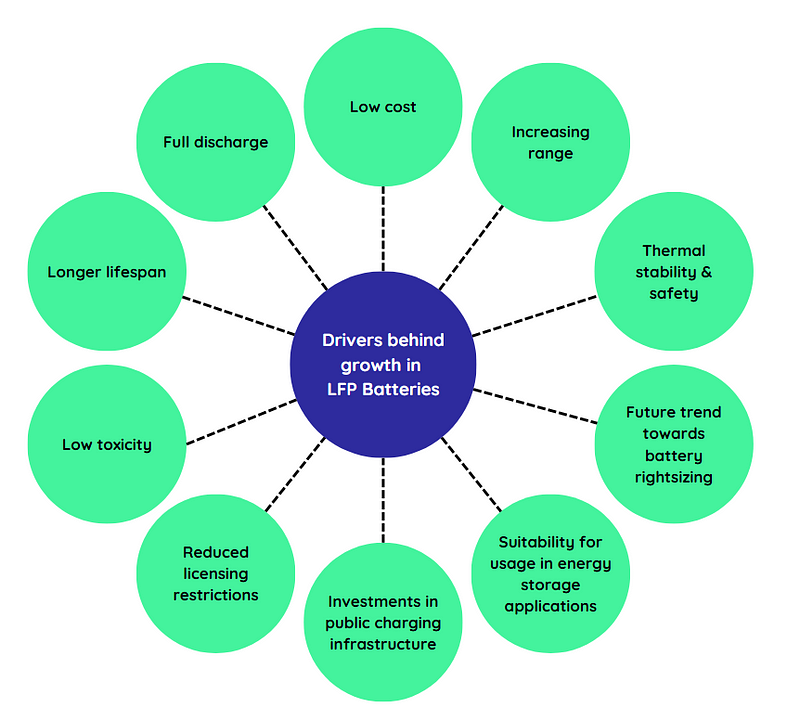
Drivers behind the growth in LFP battery demand
China has long dominated the LFP market, leveraging decades of technological refinement and economies of scale. But as global demand rises, new regional markets are emerging, driven by supply chain reshoring efforts and sustainability goals.
Governments worldwide are taking decisive action to strengthen domestic LFP battery supply chains with policies that actively encourage local production and create a fertile landscape for new investments.
From an investment perspective, LFP isn’t just a proven technology — it’s a high-growth opportunity. With affordability, performance, and scalability on its side, LFP is expected to be a cornerstone of the future battery landscape.
Automakers Go In on LFP
Several major vehicle manufacturers, including Tesla, Ford, Rivian, and Volkswagen are investing in LFP battery infrastructure as they steer towards this chemistry.
Tesla has been pivotal in accelerating LFP adoption, revealing that it would switch all standard-range vehicles globally to LFP batteries. This shift has created strong momentum for upstream suppliers, while Tesla’s expanding gigafactory network is scaling LFP production worldwide.
Advancements in battery design, like Cell-to-Pack and Cell-to-Body configurations, have boosted pack-level energy density, making LFP batteries more suitable for larger vehicles. These innovations help balance energy density trade-offs while keeping costs low, reinforcing LFP’s appeal in the EV market.
In 2023, LFP batteries were under the hood of over 40% of electric vehicles worldwide. Some estimates even suggest that in 2024, LFP made up 59% of all battery cell production globally. That’s a huge leap, showing just how fast this chemistry is taking over.
As EV sales rise, the reliance on LFP batteries is growing. This underscores an urgent need for additional suppliers of LFP cathode materials and batteries in the EU and North America. Investing in local production capacity not only mitigates supply chain risks but also positions investors to capture market share in an industry experiencing growth.
Beyond EVs: LFP’s Role in Stationary Energy Storage
The stationary storage sector is rapidly emerging as a key growth area, diversifying the customer base and extending LFP’s reach beyond transportation.
As renewable energy adoption accelerates, the demand for reliable grid stability is growing and this is where LFP stands out. Compared to NMC batteries, LFP offers superior longevity, often exceeding 3,000 charge cycles.
Combined with its long cycle life, strong safety profile, and cost-effectiveness, LFP has become the preferred choice for commercial and industrial (C&I) energy storage systems (ESS).
Major tech and infrastructure players are already integrating LFP batteries into data centre UPS (uninterruptible power supply) systems, a sector expected to grow rapidly with advancements in AI and high-performance computing. Today, LFP batteries account for over 80% of the Li-ion ESS market.
Elon Musk has been vocal about LFP’s advantages for stationary storage, noting that energy density is less critical for fixed installations. In his words:
Tesla is transitioning its Powerwall residential energy storage system to LFP chemistry.
Powering the Growth: How LFP is Made
Carbothermal Reduction (CTR) is the workhorse of LFP production, accounting for up to 90% of total output.
Its dominance isn’t by chance, CTR’s simplicity, scalability, and cost-effectiveness have made it the industry standard for large-scale manufacturing.
Industry leaders are constantly refining CTR to cut costs and reduce energy consumption, all while maintaining high product quality.
Advances in process control, energy recovery systems, and raw material utilisation are driving these improvements, ensuring CTR remains an evolving cornerstone of LFP manufacturing.
Some other notable innovations include:
- Microwave Processing:
For faster and more uniform heating, reducing both processing time and energy consumption. - Precursor Modification:
Using specific carbon sources or polymers can lower the required reduction temperature, improving cost efficiency. - Nanocomposite Formation:
Integrating additives like graphene nanosheets enhances electrochemical properties, improving conductivity and charge retention.
Beyond CTR, various alternative synthesis methods, each with advantages, but none yet widely commercialised.
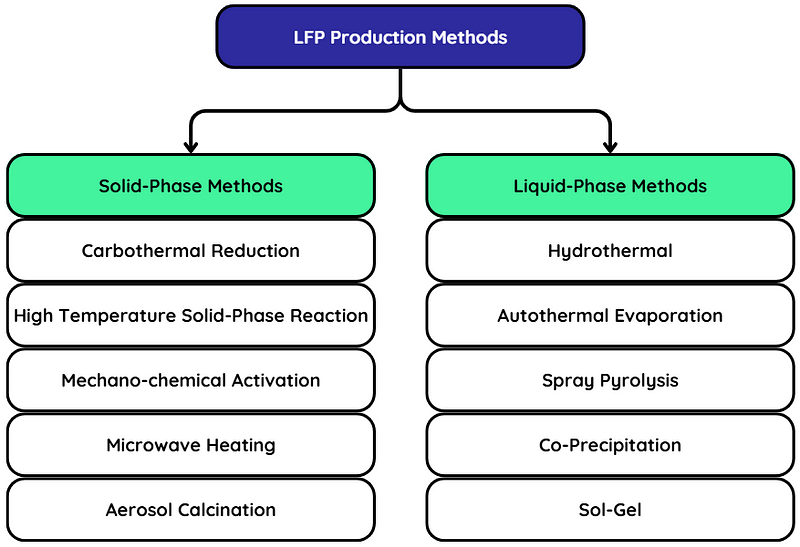
Their biggest hurdles are cost, process complexity, and product quality. Many methods, especially liquid-phase techniques, rely on intricate chemical processes and strict controls, making them harder to scale than CTR.
Still, some offer potential benefits that could disrupt the LFP landscape:
- Better Material Properties: Sol-gel and co-precipitation precisely control particle size, morphology, and composition, boosting electrochemical performance, tap density, and rate capability.
- Lower Processing Temperatures: Microwave heating and mechano-chemical activation could cut energy use and processing time versus high-temperature CTR, lowering costs.
- New Material Designs: Aerosol calcination enables composite materials and coatings for advanced LFP architectures with better conductivity and cycle life.
Ultimately, the future of these alternative synthesis methods hinges on overcoming scalability and cost challenges through material and process innovations and engineering.
China’s Lead and Western Catch-Up
China dominates LFP production, supplying nearly 100% of the global output. However, Europe, North America, and other regions are gearing up to expand their own domestic production.
With this growth, the industry is on track to reach 2,000 kilotonnes of LFP production by 2030.
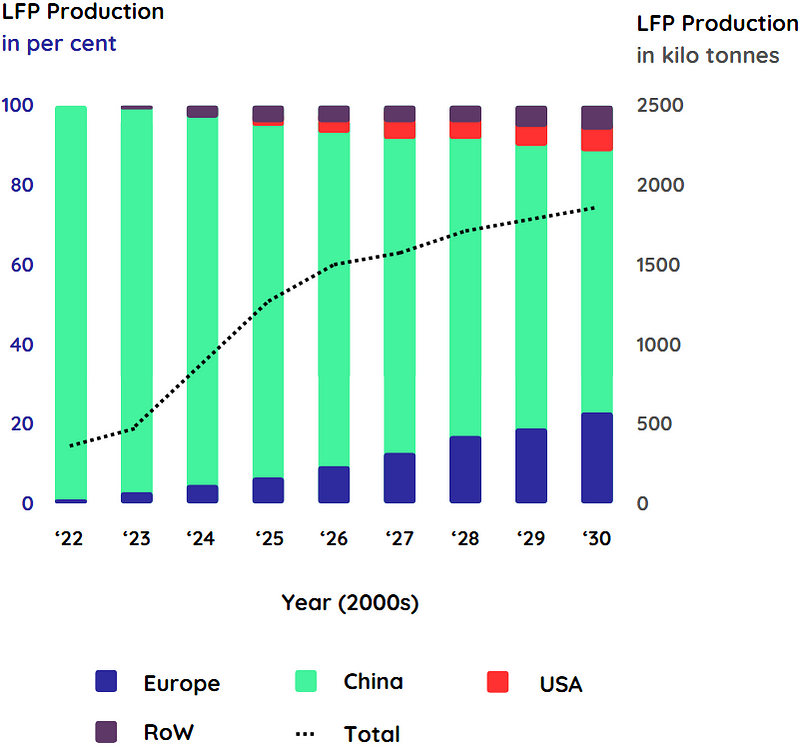
Estimated global LFP production capacity from 2022 to 2030
Source: Fraunhofer ISI
One of the biggest challenges for Europe and North America is closing the gap between their rapidly growing battery cell production capacity and their limited ability to manufacture cathode-active materials locally.
North America faces an 87% cathode material deficit, while Europe’s shortfall is even more severe at 93%. This heavy reliance on Chinese-made LFP underscores a critical vulnerability in supply chain security.
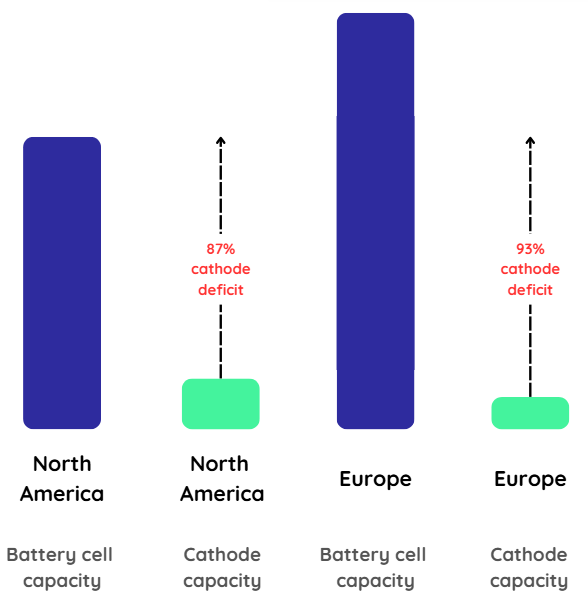
Deficit in cathode capacity in Europe and North America vs battery cell capacity
Source: Volta Foundation
To close this gap, governments are rolling out aggressive industrial policies.
In the US, the Inflation Reduction Act (IRA) offers strong incentives for domestic battery production, helping to reduce reliance on imported cathode materials. A prime example is ICL Group, which secured a $197 million grant from the U.S. Department of Energy to build a 30,000-ton-per-year LFP cathode factory in Saint Louis, Missouri.
However, with IRA funding facing potential freezes under the Trump administration, alongside shifting geopolitical priorities and growing international tensions, alternative pathways may emerge. The U.S. Department of Defense (DOD) could play a critical role in supporting onshoring efforts, opening new opportunities for startups to access both energy and national security funding streams.
China’s CTR Blueprint: A Model for Western Markets
China’s dominance in CTR serves as a blueprint for scalable, cost-effective solid-phase production for Western markets to emulate and build upon.
CTR has proven its maturity and efficiency at a gigafactory scale. Compared to liquid-phase techniques, which play a niche role in LFP manufacturing,
As a result, Western markets are driving innovation primarily through solid-phase production, with start-ups like Redoxion, NanoOne, Mitra Chem, Western CAM and HitNano.
Collectively, these companies aim to overcome the cathode deficits required to support projected battery capacity in Western markets.
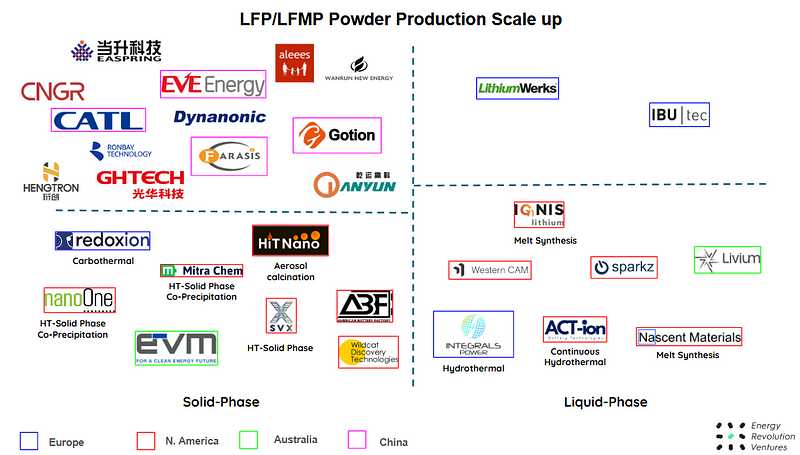
This diagram is intended to provide a high-level landscape and is not an exhaustive representation of all companies operating in this sector.
As production scales outside of China, Western players have a unique opportunity to build a domestic supply chain, reducing dependencies while keeping pace with rising global demand for LFP batteries.
Tariffs & Trade Barriers: The Push for Domestic Production
In September 2024, the U.S. hit Chinese-made lithium-ion EV batteries with a hefty 25% tariff, sending shockwaves through the auto and energy storage industries. Practically overnight, costs spiked for American automakers and battery buyers, intensifying the urgency to ramp up domestic production. The message is clear: companies can no longer afford to lean so heavily on Chinese supply chains.
China hit back in December 2024, cutting export tax rebates on batteries from 13% to 9%, effectively raising costs for Chinese exporters and international buyers. This adjustment further incentivises global manufacturers to explore alternative suppliers, reinforcing the shift toward regionalised battery production.
January 2025 brought another jolt to the battery industry. The U.S. Department of Defense officially labelled CATL, China’s largest battery maker, as a company with alleged ties to the Chinese military. While this designation doesn’t come with immediate restrictions, it casts a long shadow over the future. Could sanctions be next? The uncertainty alone can make automakers and energy players rethink their supply chain strategies, adding another twist to an already shifting global battery landscape.
Beyond tariffs and trade policies, China is moving to tighten control over critical battery manufacturing technologies, specifically focusing on producing High Compaction Density (HCD) LFP.
HCD LFP is now a game-changer in battery manufacturing, enabling higher pressed densities, a critical factor for maximising volumetric energy capacity. Chinese manufacturers have raised the bar, surpassing 2.6 g/cc and setting a new industry benchmark. This is a vital competitive advantage. To stay in the game, global players can’t just match these numbers — they’ll need to push beyond them.
HCD LFP, or “4th gen LFP,” is crucial for enhancing energy density and enabling faster charging capabilities by optimising the balance between performance and electrode thickness.
China’s potential restrictions on key HCD LFP technology could tighten supply chains, making it harder for non-Chinese manufacturers to compete. Western high-temperature solid-phase processes already lag in achieving similar densities.
Producing HCD LFP requires moving from single-sintering to a more complex double-sintering process, increasing both time and costs and potentially slowing LFP price declines. This temporary price shift could give start-ups a window to optimise and develop cost-effective methods before HCD LFP scales to match standard LFP economics.
Breaking China’s Grip on LFP: How the West Can Build a Competitive Supply Chain
One of CTR’s biggest advantages is its flexibility in raw material selection:
- Lithium Sources → Can use lithium carbonate (Li₂CO₃) or lithium hydroxide (LiOH).
- Iron Sources → Can source from iron oxide (Fe₂O₃) or iron sulfate (FeSO₄).
- Carbon Sources → Can utilize glucose, sucrose, or industrial carbon black.
- Phosphorus Inputs → Can be derived from phosphoric acid (H₃PO₄) or iron phosphate (FePO₄).
Raw material flexibility enables cost optimisation by using local or lower-cost feedstocks, cutting reliance on high-purity, region-specific inputs. This strengthens supply chains, allowing faster ramp-ups and greater operational stability, a crucial advantage in an era of geopolitical uncertainty.
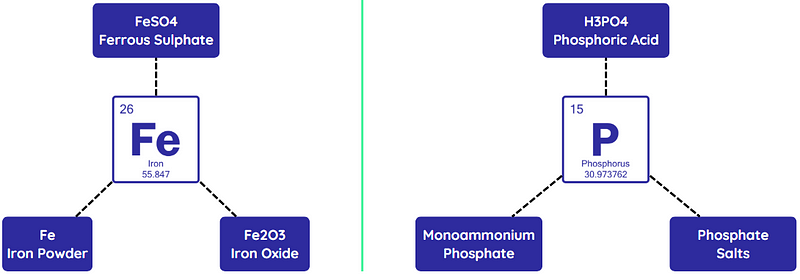
Different Iron and Phosphorus sources for LFP production
China’s Iron Grip on High Tap-Density Iron Phosphate
Despite CTR’s versatility, China dominates the production of high tap-density iron phosphate, a critical precursor material that accounts for 16% of LFP production costs.
China benefits from a unique industrial synergy:
- High tap-density iron phosphate is produced as a byproduct of the titanium industry, ensuring a low-cost, abundant supply that underpins its control over the LFP market.
- This cost-effective precursor supply chain allows China to dictate global pricing, making it difficult for Western producers to compete on cost.
Cost breakdown for LFP cathodes and iron phosphate in 2022
Source: CRU
How the West Can Overcome the Cost Barrier
The weakest link in the Western LFP supply chain lies in precursor materials, particularly the reliance on high tap-density iron phosphate. A key strategy to overcome this dependence involves leveraging commodity precursors like iron oxide.
However, current processes often require iron oxide to be combined with lithium dihydrogen phosphate (LiH₂PO₄, or LHP), which is expensive to produce and typically ends up being processed back in China, perpetuating reliance on Chinese infrastructure.
To break this cycle, Western producers must develop methods to use iron oxide or other precursors in conjunction with alternative, cost-effective commodity chemicals instead of LHP. Achieving this would create an opportunity for the West to establish a fully localised LFP supply chain.
The Investment Opportunity: Building a Resilient LFP Supply Chain
For investors, this presents a unique window of opportunity to back technologies and startups that are reshaping the global battery landscape.
As global demand for LFP accelerates, investors have the opportunity to define which regions disrupt China’s supply chain dominance.
Key areas for strategic investment include:
- Strengthening Regional Supply Chains: Developing robust, localised supply networks for LFP cathode materials to ensure long-term energy security and market stability.
- Innovating Precursor Processing: Supporting R&D in alternative precursor materials to eliminate dependence on Chinese-derived iron phosphate or LHP.
- Advancing LFP Production: Focusing on flexible raw material inputs to maximise cost efficiency and reduce supply chain vulnerabilities.
LFMP: LFP’s Younger Sibling
Building on the strengths of LFP, Lithium Iron Manganese Phosphate (LFMP) is a second generation of LFP, by adding manganese into the cathode mix to enhance electrochemical performance.
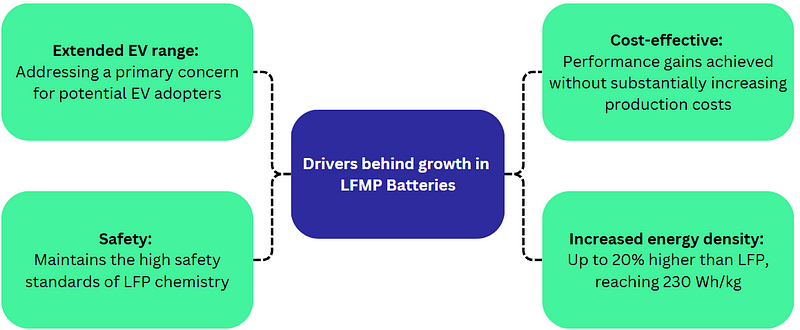
LFMP offers an attractive middle ground. It provides higher energy density than LFP, without the supply chain risks and costs of nickel- and cobalt-based batteries.
Like most younger siblings, it’s still figuring things out and has plenty of kinks to work through.
LFMP’s commercial viability hinges on overcoming key material and supply chain challenges. A high manganese composition, typically around 80%, is required and achieving the right material properties remains a hurdle, leaving room for process innovation and optimisation.
LFMP’s Technical Challenges & Innovation Opportunities
Conductivity Limitations & Impurity Sensitivity
Unlike LFP, LFMP has inherently lower electrical conductivity, behaving more like a dielectric material. This means that:
- A highly phase-pure composition is critical → Even minor impurities can trigger manganese dissolution, which can plate onto the anode and degrade performance.
- Cycle life remains a concern → While LFP boasts exceptional longevity, LFMP typically struggles beyond a few thousand cycles, with capacity fade accelerating over time.
Particle Size vs. Processability Trade-Off
To mitigate diffusion limitations, LFMP requires smaller primary particle sizes. However, this lowers tap and press densities, creating a delicate balance between performance and manufacturability.
A promising solution? Blending LFMP with LFP.
- This hybrid approach combines the strengths of both materials, striking a balance between energy density, conductivity, and cycle life.
- Manufacturers are actively experimenting with blended compositions to optimise scalability and real-world performance.
China’s Edge & The Need for Western Investment
As an evolution of LFP, LFMP can be produced using existing LFP infrastructure, giving Chinese manufacturers a major advantage. With minimal retooling, they can scale production quickly, reinforcing their dominance in the global battery supply chain.
As manganese grows in importance for next-gen LFMP batteries, China’s dominance in processing and refining remains a key factor. With established infrastructure and economies of scale, it remains the most cost-effective supplier of manganese sulphate, keeping the global supply chain heavily dependent.
Currently, there are only two manganese refineries outside China, one in Japan and one in Belgium, highlighting the global imbalance in processing capacity.
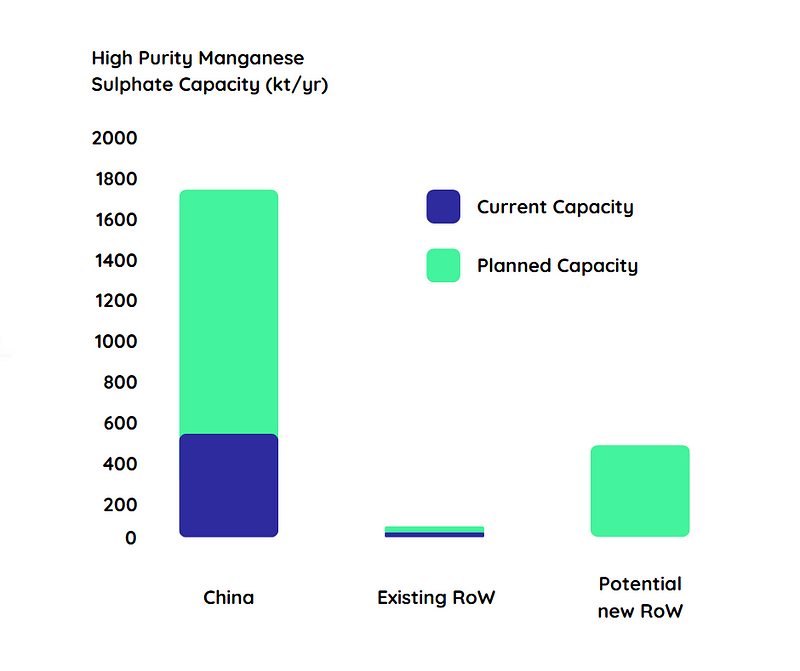
Source: CRU
Despite China’s dominance, Western markets can cut reliance on Chinese imports by developing localised supply chains. With key manganese producers in North America, Europe, Australia, and Africa, the groundwork is there for future refining expansion.
- North America → Canadian Manganese, Nevada Silver, South32, Element 25, Vibrantz
- Europe → Euro Manganese
- Australia → Black Canyon, Mn Energy, FireBird Metals
- Africa → MTC, Giyani Metals Corp (Southern Africa), Keras Resources (West Africa)
To compete with China’s dominance, Western nations must:
- Accelerate investment in manganese refining facilities to close the processing gap.
- Forge partnerships with regional producers to develop an integrated, end-to-end supply chain.
- Leverage government-backed incentives to drive localised production.
Securing manganese refining independence is now a critical step in the broader effort to strengthen Western battery supply chains.
The Road Ahead for LFP Batteries
The battery industry sits at a crossroads, shaped by protectionist policies and initiatives like the IRA. With Donald Trump’s administration imposing new tariffs on key trade partners, the landscape grows more complex. While the IRA was designed to boost domestic battery production and reduce reliance on foreign supply chains, its impact could be significantly altered under the new administration. These tariffs risk counteracting the IRA’s benefits by increasing costs for critical materials, potentially slowing the growth of clean energy sectors it was meant to accelerate.
However, this evolving scenario may also serve as a catalyst, accelerating the push for greater supply chain resilience and localised LFP manufacturing. As the battery industry navigates these shifting tides, adaptability in LFP production will be key.